Icy Elements from the Early Solar System
- Webmaster
- May 22, 2021
- 8 min read
Updated: May 29, 2021
Element Nucleosynthesis
Elements; the building blocks of what makes up: us, the Earth we live on, and the entire Universe. They shape everything that happens throughout each day of our lives, from the copper supplying our energy; to the lithium storing it; and the oxygen we breathe.
However, they haven’t been here forever. Through processes of nucleosynthesis (creating new nuclei of atoms from the protons and neutrons that make them up) which have happened and are happening throughout the Universe, we are able to live the way we do. These processes include: big bang fusion, cosmic ray fission, neutron star mergers, explosions, and dying low mass stars (as well as many other methods, including in particle accelerators here on Earth) [1].
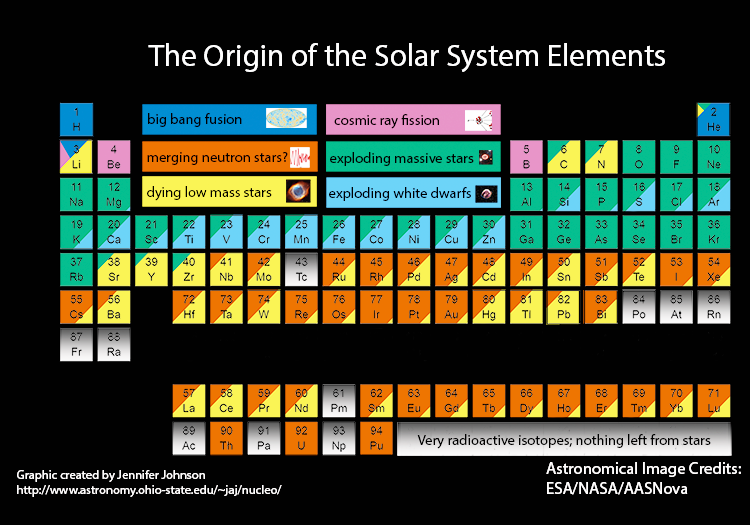
Colour coded periodic table showing the origins of elements in the Solar System.
Credit: Jennifer Johnson, CC BY-SA 4.0
The Big Bang
We start our elementary journey at the beginning of time; the beginning of everything: the Big Bang.
An event so powerful the effects are still seen today in the form of the Cosmic Microwave Background Radiation (the remains of the Big Bang, emanating from the voids of space at the freezing 2.7 kelvin: 2.7ºC above the coldest possible temperature [2]), the Big Bang emitted so much energy that it’s no wonder some crazy things went on back then.
Over the first 3 minutes after the beginning of the Universe (starting at the Universe’s spritely age of 1 second), the first elements came into being: hydrogen (specifically deuterium -a neutron and a proton- and tritium -two neutrons and a proton-), helium (helium-3 and helium-4 to be precise), lithium (lithium-7) and beryllium (beryllium-7). And they all came into being through fusion: the smashing together of particles and atoms to make atoms of elements with higher masses.
In fact some of the deuterium, helium and lithium from the Big Bang (known as primordial elements) is still around in the Universe today, as a 13.7 billion year old relic of the Universe’s past. However, the same cannot be said for the beryllium and tritium, with half lives (the time it takes for an atom to decay by half into another element) of just 53 days and 12 years respectively.
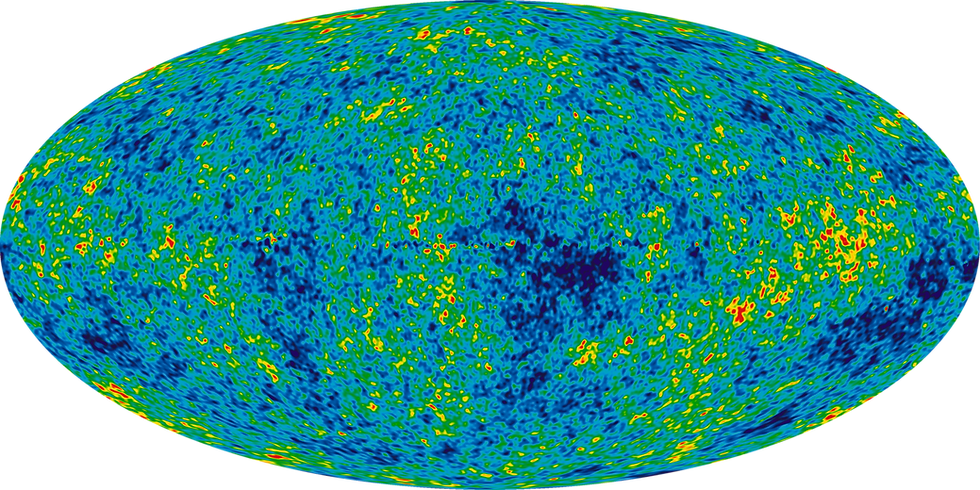
The Cosmic Microwave Background Radiation (CMBR)
Credit: NASA/WMAP Science Team
Cosmic Rays
The Universe is therefore full of hydrogen and helium… but not lithium. The third element in the periodic table and one of the three to have braved 13.7 billion years of time to be here today is not a very abundant element, yet, generally speaking, the lower (the less protons) in the periodic table an element is, the more of it there is. So why is lithium an exception?
The real amount of lithium made in the Big Bang was a feeble 0.0000001% (a ten millionth of a percent) of the Universe’s elements at the time (as opposed to the ~75% hydrogen and ~25% helium). In other methods all involving stars (which we’ll talk about later), lithium (along with beryllium and boron) also can’t be created in the same quantities we see it in today. Instead, enter cosmic ray spallation [4].
When cosmic rays (usually high energy neutrons) collide with an atom’s nucleus, multiple particles from that nucleus are released, which then begin a chain reaction of more and more collisions and emissions of particles, known as a cosmic ray cascade. And this isn’t even the best part!
The particles, or spalls, that fly out from the atoms which have had a collision are then absorbed into an atom, creating a new isotope (a new number of neutrons in the atom) or even a new element (a new number of protons in the atom), leading to lithium, beryllium and boron being formed. [5][6][1]. There’s only one problem though. Lithium-6 is the isotope made by cosmic ray spallation; not lithium-7: the most common type, and also the type made in the Big Bang [7].
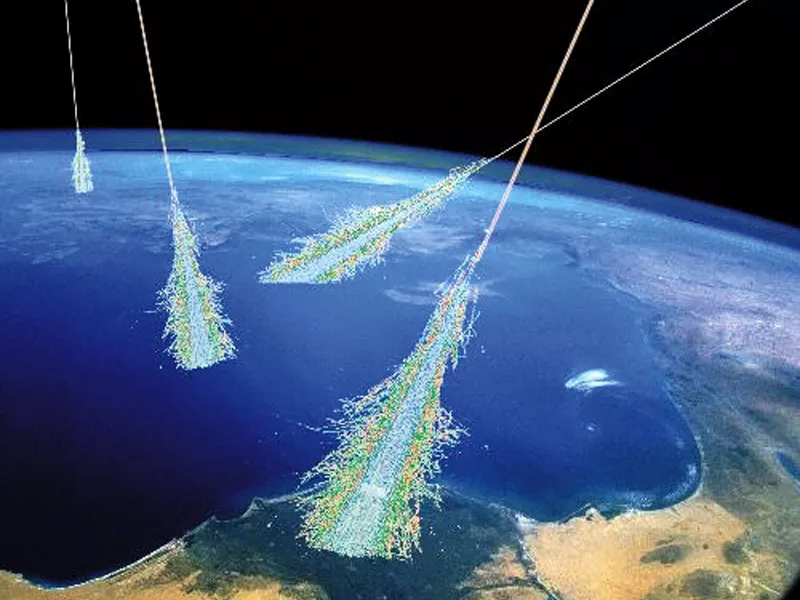
Artist conception of showers of cosmic rays passing through Earth's atmosphere.
Credit: Simon Swordy (U. Chicago), NASA
Explosions and Mergers
Jumping to the much larger scale, r-process nucleosynthesis is the source of many useful elements we see in the Universe, and on Earth, today, including the likes of: gold, silver and iodine (all useful to us in many ways) [7]. And there are two ways r-process nucleosynthesis could occur: neutron star mergers and supernovae [8].
Supernovae are by far the most famous method of the two. As massive stars (greater than 9 times our Sun’s mass) come to the end of their lives as burning giants, they begin to collapse at around a quarter of the speed of light at their fastest collapse, before bouncing outwards, splurging neutrinos and gases into the surrounding environment in a shockwave (moving at up to a tenth of the speed of light), beginning nuclear fusion, whereby atoms fuse to make heavier and heavier elements.
This then breaks out into a full blown supernova, where the star shines at around 10 billion times the Sun’s brightness (brighter than whole galaxies) to leave a core behind. However, it’s the hot bright phase that is the most important: a flurry of rapid neutron captures (the ‘r’ in ‘r-process’ is for ‘rapid’) occur, where neutrons enter atoms to turn them into larger and larger isotopes of their element. However, this only happens to a point, since the nucleus of the atoms (what is receiving the neutrons) reach neutron drip.
This is where the forces holding the neutrons together in the atom’s nucleus stars to decrease and become negative, stopping any more neutrons from entering. Beta-minus decay then occurs, where the neutrons change into protons, both increasing the number of neutrons that can enter the atom (since there’s now less negative charge because of the proton’s positive charge) and increasing the atom’s atomic number, creating heavier and heavier elements [9][10].
This isn’t it for the massive stars though! Instead, those that are on the less massive range turn into neutron stars: stars made of protons and electrons which are crushed together so much they turn into neutrons. In fact, they’re so compressed just one sugar cube sized piece would weigh as much as 1 trillion kilograms (or one seventh the mass of Mount Everest [11][12]) [11]. And this abundance of neutrons can only mean one thing: rapid neutron capture.
When two neutron stars merge (having pulled each other closer by their immense gravitational attraction -I know, so romantic!) the r-process kicks in, creating many more useful elements and throwing them out into the Universe to become, along with what's produced in Supernovae, what’s in our smartphones and what’s in our sushi [13].
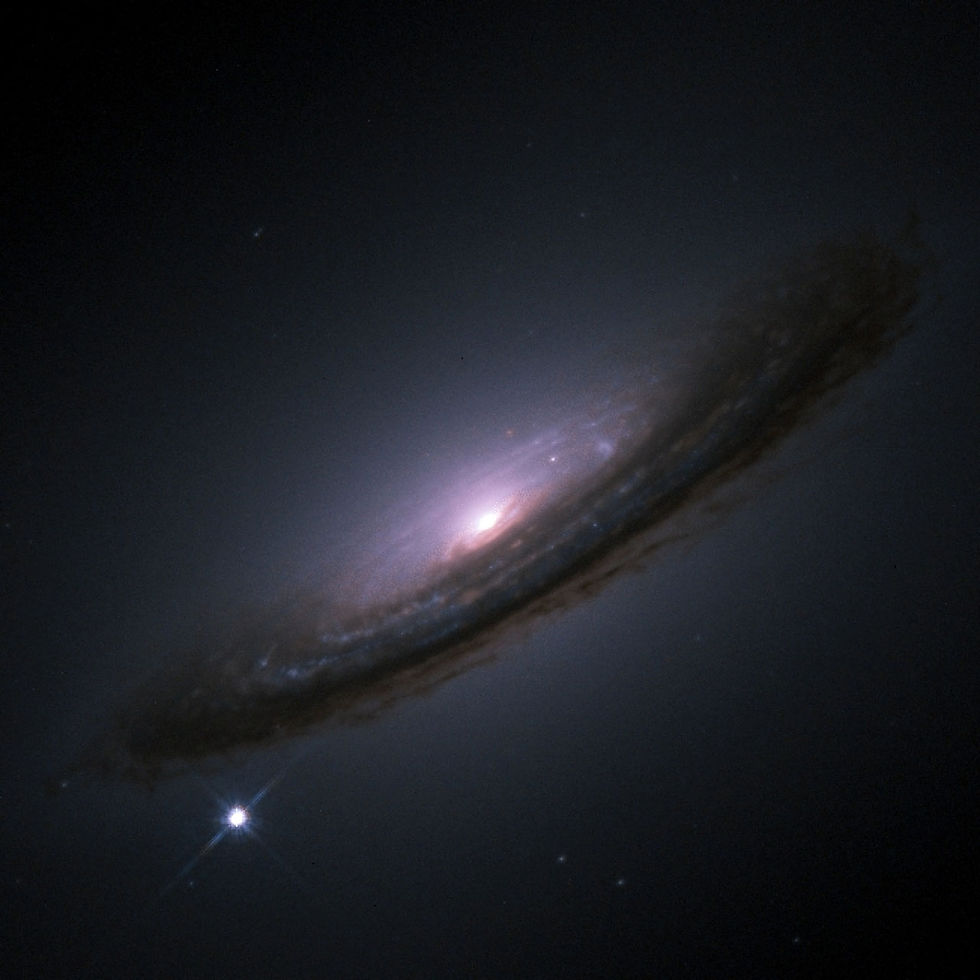
Type 1a Supernova SN 1994D (bottom left) near galaxy NGC 4526
Credit: NASA/ESA/ The Hubble Key Project Team/ the High-Z Supernova Search Team,
A Bit Closer to Home
Low mass stars (such as our Sun), unlike the massive stars that have their destiny making high mass elements, have more humble, yet arguably more important, destinies. All these low mass stars burn through hydrogen but, unlike the modern fuel source hydrogen has come to be here on Earth, they fuse it to get energy; a lot of energy!
Apart from energy though, this fusion of hydrogen atoms forms helium, leading to hydrogen and helium being the most abundant elements in the Universe (though not on Earth, since they are very good at escaping due to their low masses). Once all the hydrogen has been eaten up though, a star’s next meal will be the heavier elements, all the way up to iron [14].
Why stop at iron though? This is because it takes more energy to fuse than what the fusion process produces, resulting in there being not enough energy to sustain a star, leading to its collapse. However, our Sun, along with many other low mass stars (less than 9 times the mass of the Sun) will stop much earlier (somewhere around carbon), forming a red giant [15][16].
That said, they may have a few more elements in them, forming by the s-process (’s’ for ‘slow’). This process involves neutrons which are emitted (or liberated) from neutron rich atoms like carbon-13 and neon-22, before being captured by other atoms and turning into protons (through beta-minus decay) to create new elements (up to lead) This is the slow process though, taking place over thousands of years instead of the seconds that the r-process takes [17][18].
Eventually, this excess mass accumulating around the red giant is blown away in a planetary nebula, leading to a white dwarf. This white dwarf is then the source of the majority of the lithium in the Universe (25%) due to novae: the emission of a bit of the white dwarf due to the absorption of some hydrogen from a nearby star, leading to the white dwarf emitting a lithium producing isotope in the process [19].
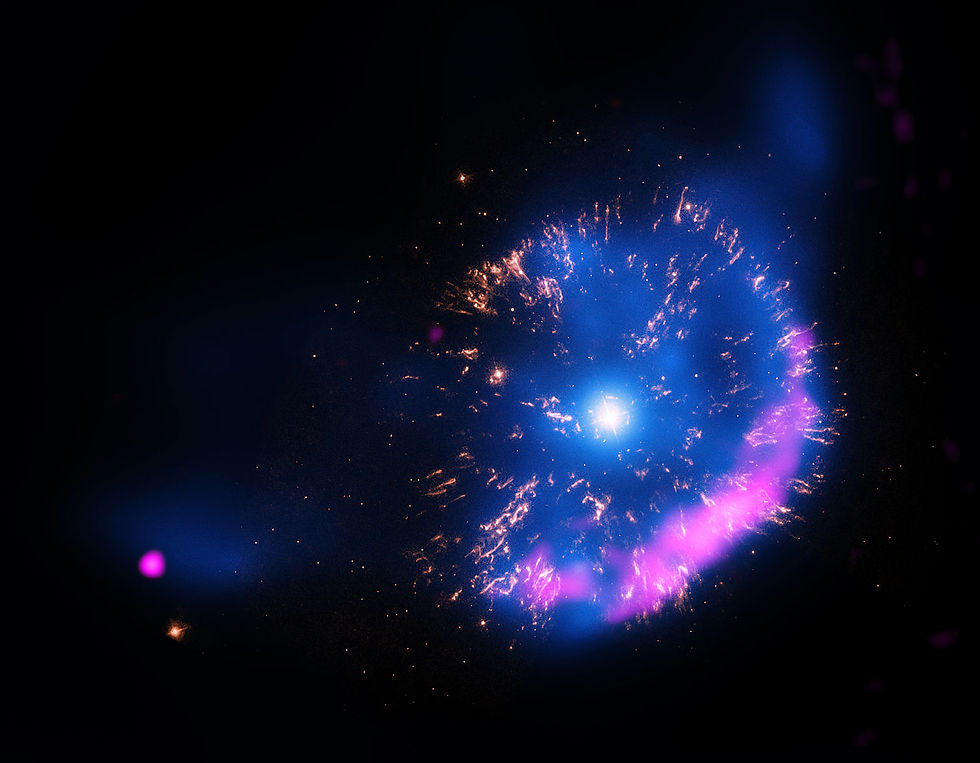
GK Persei Nova Remnant.
Credit: X-ray: NASA/CXC/RIKEN/D.Takei et al; Optical: NASA/STScI; Radio: NRAO/VLA
Cold Metal
Why have I been going on about the formation of elements though? It all comes down to the recent news which has come from ESO (the European Southern Observatory), who have provided the data for a study by a Belgian team of scientists who discovered iron and nickel vapours on the surface of comet C/2016 R2 (PANSTARRS), 480 million kilometres from the Sun, where they’ve never been observed before due to the expectation that only hot environments would provide the correct temperatures for the vaporisation of these heavy metals.
Iron, with atomic number 26, and nickel, with atomic number 28, are inherently formed in different ways and therefore, most importantly, in different quantities. So, you’d expect lots of iron and a bit less nickel (10 times the amount of iron to be precise). However, through spectroscopy, the chemical signatures of iron and nickel were found in equal amounts!
What’s more, the interstellar comet 2I/Borisov is also showing similar amount of these two elements, therefore hinting that both comets were formed in similar environments, suggesting that what we see in our Solar System is a good template for what’s seen in other parts of the galaxy, and maybe even the Universe; whilst also bringing up the question of why there is this equal amount of nickel and iron seen on comets [20].

Heavy metal detection in the atmosphere of comet C/2016 R2
Credit: ESO/L. Calçada, SPECULOOS Team/E. Jehin, Manfroid et al., CC BY 4.0
by George Abraham, ADAS member.
#Element #Nucleosynthesis #Comet #Spectroscopy #Neutrino #Neutron #Proton #Supernova #NeutronStar #CosmicRay #BigBang
Click here for the previous news article
Click here for the next news article
Click here to watch an animation of the heavy metal composition of comet C/2016 R2 (PANSTARRS)
Click here to find out where comet 2I/Borisov is on its journey out of the Solar System, and here to look where comet C/2016 R2 (PANSTARRS) is on its orbit
References
"The Origin of Elements". The Ohio State University. Archived from the original on 22nd May 2021.
"Lecture 31: The Cosmic Microwave Background Radiation". University of Alberta. Archived from the original on 22nd May 2021.
"How the Big Bang forged the first elements". Astronomy.com. Archived from the original on 22nd May 2021.
"This is Why Three of the Lightest Elements are so Cosmically Rare". Forbes. Archived from the original on 22nd May 2021.
"Cosmic Rays". AntarcticGalciers.org. Archived from the original on 22nd May 2021.
"Spallation and the creation of the elements lithium, beryllium and boron". YouTube. Archived from the original on 22nd May 2021.
"Origin of the Elements in the Solar System". Science Blog from the SDSS. Archived from the original on 22nd May 2021.
"Colliding Neutron Stars as the Source of Heavy Elements". AAS Nova. Archived from the original on 22nd May 2021.
"Lecture 18: Supernovae". The Ohio State University. Archived from the original on 22nd April 2021.
"The R-Process". Caltech. Archived from the original on 22nd May 2021.
"Neutron Stars". NASA. Archived from the original on 22nd May 2021.
"Estimating the Mass of Mount Everest". Viktor Plamenov. Archived from the original on 22nd May 2021.
"DOE Explains... Nucleosynthesis". US Department of Energy. Archived from the original on 22nd May 2021.
"Stars & Their Energy Sources". CSIRO. Archived from the original on 22nd May 2021.
"What Happens when Stars Produce Iron". Futurism. Archived from the original on 22nd May 2021.
"s-process". David Darling. Archived from the original on 22nd May 2021.
"A New Site for the s-Process". AAS Nova. Archived from the original on 22nd May 2021.
"Most Lithium in the Universe is Forged in Exploding Stars". Smithsonian Magazine. Archived from the original on 22nd May 2021.
"Heavy metal vapours unexpectedly found in comets throughout our Solar System – and beyond". ESO. Archived from the original on 22nd May 2021.
Comments