Mapping the Invisible
- Webmaster
- May 29, 2021
- 6 min read
Updated: Jun 27, 2021
Let’s Start at the Very Beginning…
13.7 billion years ago the Big Bang happened: the birth of the Universe. The building blocks of nuclei (protons and neutrons) formed, before combining to form the nuclei of the first elements of the Universe. However, the temperature and pressure of the Universe was so high atoms couldn’t form, since electrons weren’t able to combine with the nuclei. Instead, a hot ionised plasma fog was formed, scattering radiation with each forced separation of electrons from a nucleus.
This then created an impenetrable wall of radiation which lasted for 380,000 years, before it was then cool enough for electrons to finally meet with nuclei to form neutral atoms (the epoch of recombination - the biggest reunion in the history of the Universe, it could be said). This radiation left a mark on the Universe, still visible to this day in the form of the Cosmic Microwave Background Radiation (CMBR) [1][2].
The CMBR is the key to understanding everything that comes later. Hidden within it are seemingly random fluctuations in the amount of radiation emitted: the weaker the radiation, the more the photons had to fight their way through the impenetrable fog and loose energy because of it, meaning weaker radiation shows denser areas of matter.
And these minute fluctuations in density, quantum fluctuations, were formed in random incidences in the first event of rapid inflation in the 0.00000000000000000000000000000001 seconds (that’s 32 zeros: a one hundred-nonillionth), with an increase in size by a factor of 1,000,000,000,000,000,000,000,000,000,000 (1 with 30 zeros: a nonillion). This increase dramatically amplified the small quantum fluctuations to an enormous scale, leading to denser areas of matter and sparser areas of matter. The denser areas then became denser and denser due to gravitational attraction, leading to what we see today: the cosmic web [3].
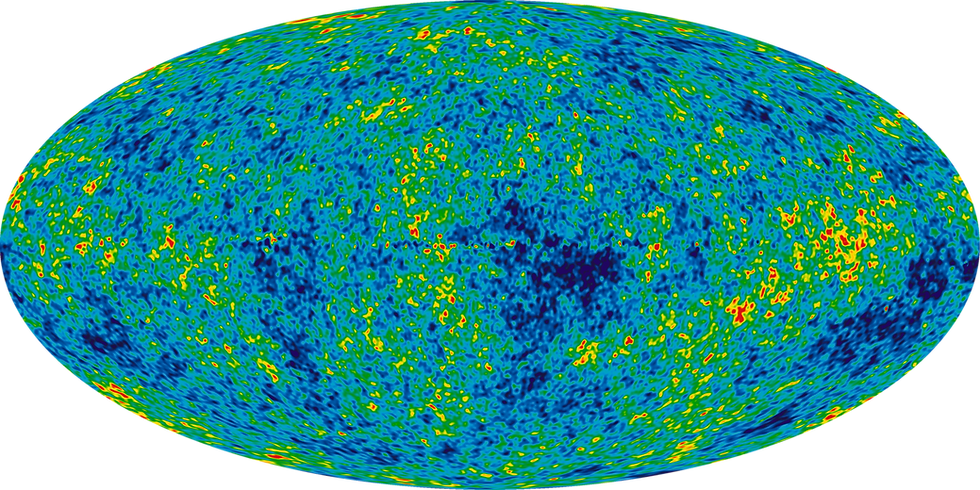
The Cosmic Microwave Background Radiation (CMBR)
Credit: NASA/WMAP Science Team
Universe Wide Web
Over the next 13.7 billion years or so, these quantum fluctuations kept on amplifying, ordering objects, such as galaxies, into thick long strings (filaments), creating voids of near-empty space (where the laws of physics may not be the same as here on Earth). These filaments then condensed into large galaxy clusters, which are where galaxy groups (such as the Local Group, made of galaxies such as the Milky Way, Andromeda, and Triangulum) congregate, making up thousands of galaxies with a collective mass of between 100 trillion and 1 quadrillion times the mass of our Sun, and around 30 million light years across [4].
As time goes on, the filaments will feed into the galaxy clusters, forming galaxy superclusters, at more than 100 million light years across. Eventually, these superclusters will become islands of galaxies, separated by voids of very low density, containing isolated stars and galaxies[4][5]
There’s one problem though: there’s not enough stuff to produce that much of a pull to create such structures.
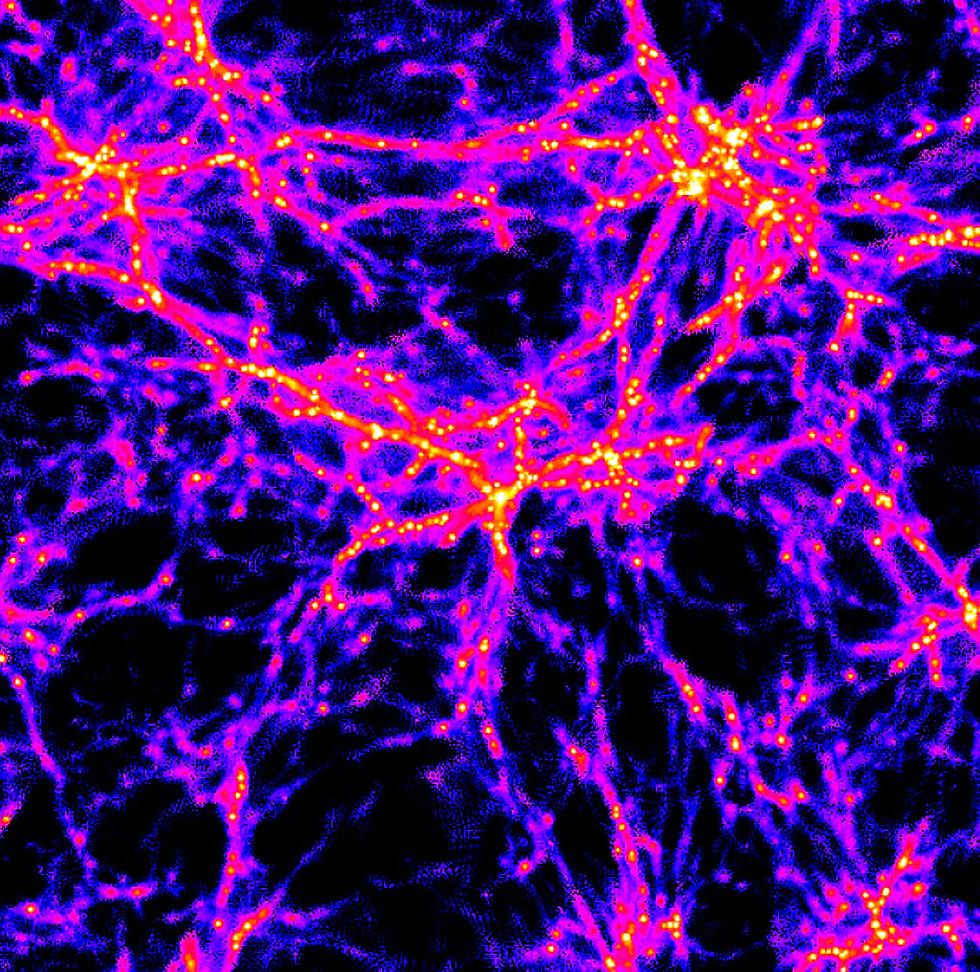
Computer simulation of the 3 Universe, at 3 billion years old, where filaments and voids are forming, along with areas of slightly higher densities: galaxy clusters.
Credit: ESO, CC BY 4.0
The Invisible Web
The answer lies in dark matter. Making up 27% of the Universe and 80% of matter in the Universe, dark matter creates a skeleton for the cosmic web to form around. And around galaxies dark matter clusters further, creating dark halos (the Milky Way has a halo which is 300,000 light years across, and possibly even further -the Milky Way itself is only 100,000 light years across), linking galaxies together gravitationally [6][7][8][9][10].
But, if it’s invisible, how come we’ve got pictures of it? There are a number of methods.

Chandra X-Ray Image of Abell 2029: a cloud of hot gas where multiple galaxies lie.
Credit: NASA/CXC/UCI/A.Lewis et al
What’s not there?
The first method is to observe how the stars and gas within galaxies move about. If the amount of matter which can be seen doesn’t account for how the stars and gas move, then there’s something at play, and that something could be dark matter. The higher the speed of motion, the greater the mass there is to pull those objects around the galaxy [8].
This can then be applied to much much larger scales, such as galaxy groups or clusters. If the speed of the galaxies that make up the groups and clusters are so high that, theoretically, they should fly out of the group or cluster, it could be dark matter that’s keeping everything together. In fact, this particular theory explains how the gravitational pull between the nearby Andromeda Galaxy and the Milky Way is so strong as to pull them together to then collide in 3 billion years [8][10].
Then there’s the observations of high energy X-rays, which are emitted from gases found throughout galaxy clusters. The gas physically shouldn’t be emitting that much energy, sometimes exceeding a million Kelvin (around a million ºC), but instead drifting away from the galaxy clusters and evaporating. However, a dark source of extra gravitational pull seems to be holding this gas together: dark matter [8][11].
And then there’s gravitational lensing: an effect whereby the light from something behind a place with a strong gravitational field distorts and magnifies that light behind, sometimes even creating multiple images of the same thing. The are various effects involving this, including rings, crosses and arcs. If there’s no obvious source of mass distorting the light, one source could be dark matter [12][13][14].

The Einstein Cross: four images of a distant quasar, being an effect of the gravitational lensing of a nearby galaxy. Credit: NASA/ESA/STScI
The Map
Using the gravitational lensing technique, along with some handy artificial intelligence to look at the 100 million galaxies in the data set, the Dark Energy Survey (an international collaboration to survey the night sky and uncover the secrets of dark energy and dark matter) were able to produce a map of the dark matter in a quarter of the southern hemisphere’s sky.
There are two key findings that were produced from the paper behind this magnificent map (the largest dark matter map made using gravitational lensing). First is that the dark matter in our Universe is smoother than computer simulations expect (using equations from the standard model: the model from Einstein’s theory of general relativity), suggesting there may be something missing in the maths.
The next is that we now know exactly where the voids are in that part of the sky (since they’re more obvious when looking specifically at the layout of dark matter), meaning we can point our telescopes there to see if physics seems to work any differently, and if so, how [9][15][16][17][18].

Map of the optical Gaia sky overlaid with the map of dark matter, showing: filaments, voids, and halos (where galaxy clusters lie)
Credit: N. Jeffrey/Dark Energy Survey Collaboration
Hopefully this discovery, along with many more to come, will open the horizons for more meaningful observations of the Universe, so we can get a broader view of how our Universe functions and find out what dark matter actually is.
by George Abraham, ADAS member.
#DarkMatter #CMBR #CosmicWeb #Halo #DES #GravitationalLensing #GeneralRelativity #StandardModel #Filament #GalaxyGroup #GalaxyCluster
Click here for the previous news article
Click here for the next news article
Click here to look at the paper behind the map
Click here to watch the video to learn more about the papers released by DES, and the enormity of the project, and here for a more in-depth view
Click here to look at the other papers produced in the DES Year 3 cosmology analysis
Click here to look at galaxy cluster IDCS 1426, here to look at the Einstein Cross and here to look at the sheer number of galaxies in Abel 2029, all on ESA Sky
Click here for an activity by JPL to simulate how we detect dark matter in the Universe.
References
"How did the first element form after the Big Bang". Astronomy Magazine. Archived from the original on 29th May 2021.
"Cosmic Microwave Background". Swinburne Astronomy Online. Archived from the original on 29th May 2021.
"The cosmic microwave background and inflation". ESA. Archived from the original on 29th May 2021.
"Galaxy Cluster". Universe Today. Archived from the original on 29th May 2021.
"'We Don't Planet' Episode 7: The Vast Cosmic Web". Space.com. Archived from the original on 29th May 2021.
"Dark matter". CERN. Archived from the original on 29th May 2021.
"New Map of Local Dark Matter Reveals 'Bridges' between Galaxies". Sci News. Archived from the original on 29th May 2021.
"Dark Halo". Swinburne Astronomy Online. Archived from the original on 29th May 2021.
"New dark matter map reveals cosmic mystery". BBC News. Archived from the original on 29th May 2021.
"Hyperfast Star Was Booted From Milky Way". NASA. Archived from the original on 29th May 2021.
"Study Suggests Andromeda Crashed into the Milky Way 10 Billion Years Ago". SciTechDaily. Archived from the original on 29th May 2021.
"7 Incredible Discoveries from two Decades of X-Rays". Sky & Telescope. Archived from the original on 29th May 2021.
"Gravitational Lensing". Hubblesite. Archived from the original on 29th May 2021.
"Ask Astro: If dark matter is invisible, then how do we detect it?". Astronomy Magazine. Archived from the original on 29th May 2021.
"Astronomers have created the largest ever map of dark matter". NewScientist. Archived from the original on 29th May 2021.
"Astronomers create largest map of the universe's dark matter". The Guardian. Archived from the original on 29th May 2021.
"Overview". The Dark Energy Survey. Archived from the original on 29th May 2021.
"DES Year 3 Cosmology Results: Papers". The Dark Energy Survey. Archived from the original on 29th May 2021.
Commenti